We have performed linear and nonlinear finite element analysis of a series
of mechanistic models of the fingerpad under a variety of mechanical stimuli
(Srinivasan and Dandekar, 1992; 1996; Dandekar and Srinivasan, 1994; 1995;
Dandekar and Srinivasan, 1996). The models range from a semi-infinite
medium to a 3D model based on the actual finger geometry, and composed
of a homogeneous elastic material, a thick elastic shell containing a
fluid or a multilayered medium. Simulations of the mechanistic aspects
of neurophysiological experiments involving mapping of receptive fields
with single point loads, determination of spatial resolution of two-point
stimuli, and indentations by single bars as well as periodic and aperiodic
gratings have been carried out for the 2D and 3D models. We have also
solved the nonlinear contact problem of indentations by cylindrical objects
and sinusoidal step shapes. The large number of numerical calculations
needed even for the linear two dimensional models necessitated the use
of the Cray-C90 at the NSF Pittsburgh Supercomputer Center.
The results show that the model geometry has a significant influence on
the spatial distribution of the mechanical signals, and that the elastic
medium acts like a low-pass filter in causing blurring of the mechanical
signals imposed at the surface. Multilayered 3D models of monkey and human
fingertips accurately predicted the surface deformations under a line
load, experimentally observed by Srinivasan (1989). The same models predicted
the experimentally observed surface deformations under cylindrical indentors
as well. These 3D finite element models were used to simulate neurophysiological
experiments involving indentation by rectangular bars, aperiodic gratings,
cylindrical indentors and step shapes. Several strain measures at typical
mechanoreceptor locations were matched with previously obtained neurophysiological
data, so as to determine the relevant mechanical signal that causes the
receptors to respond. In all the simulations, the strain energy density
at the receptor location was found to be directly related to the static
discharge rate of the Slowly Adapting afferents. In addition, strain energy
density is a scalar that is invariant with respect to receptor orientations
and is a direct measure of the distortion of the receptor caused by loads
imposed on the skin. We have therefore hypothesized that the strain energy
density at the receptor site is the relevant stimulus to the slowly adapting
receptors.
  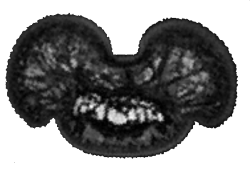
In order to further improve the spatial resolution, a three dimensional
finite element model with high mesh density near the loading region has
been developed. To model the internal geometry more accurately, we have
investigated the use of Magnetic resonance Imaging (MRI) to visualize the
internal structures of the fingerpad. In preliminary experiments we have
achieved a resolution of 60 microns/pixel. Also, to account for the dynamic
behavior of the fingertip, the models are being enhanced to include viscoelastic
effects. Once the models achieve sufficient spatial resolution and simulate
temporal effects, they can be used to generate hypotheses on how the central
nervous system might infer object shape from mechanoreceptor signals.
Click on the following links to read more in specific areas.
|
|